Exploring Cold Seeps Off Southern California
While exploring off the coast of southern California in January 2023, the Student Explorations Around Southern California: Acoustics, Paleolandscapes, and Environments at Sea (SEASCAPES) team made an exciting discovery – the first known shallow-water cold seeps in the region. Read on to learn more about these unique ocean ecosystems.
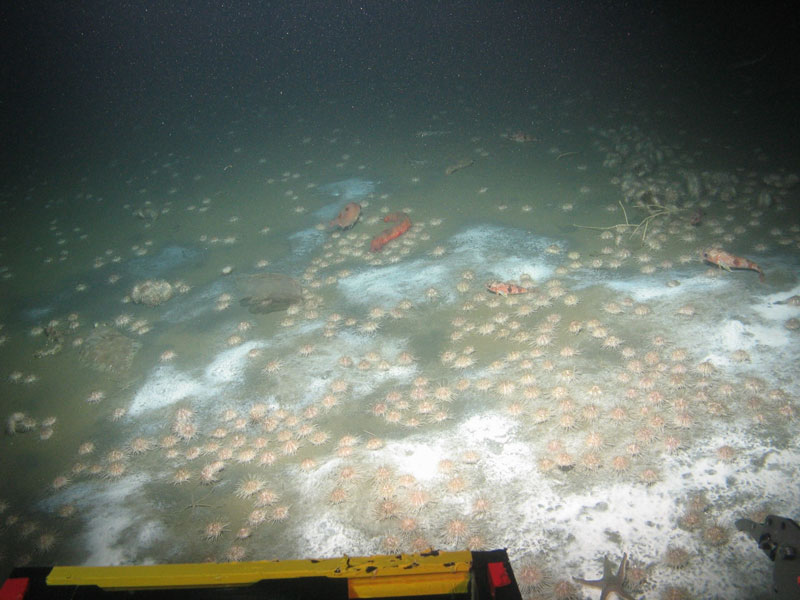
Cold seeps can be found at full ocean depths and are often associated with rich and specialized microbial and benthic animal populations that take advantage of the seeps' supply of energy-dense gases and fluids (Jessen et al., 2011; Levin et al., 2016). At cold seeps, organisms called chemotrophs or methanotrophs anaerobically oxidize methane, creating the chemical compound bicarbonate (Elvert et al., 1999; Hinrichs et al., 1999; Thiel et al., 1999). As bicarbonate is a base, its creation increases the alkalinity of pore water near cold seep sites, causing precipitation of authigenic carbonate minerals (i.e., carbonate reefs) in the shallow subsurface and the release of carbon dioxide (Baker & Burns, 1985).
The carbonate features formed at cold seep sites can provide anchor points for other forms of biodiversity on the seafloor, making previously uninhabitable regions biologically productive (Al-Zaidan et al., 2006; Van Dover & Fry, 1994). Most famously, in the deep ocean, below the euphotic zone (below 200 meters/656 feet), cold seeps can support prolific ecosystems despite the lack of light due to the presence of chemotrophs (Tunnicliffe et al., 2003). The chemotrophs provide energy (organic carbon) through chemosynthesis in lieu of photosynthesis for local marine life, but also provide habitat through deep-sea reefs.
These deep-sea ecosystems can represent discrete areas of dense biodiversity, such as tubeworms, mussels, and soft corals, in an area of otherwise low biological activity (Dando, 2010). To date, the majority of research on cold seeps and the related fauna has been focused on seeps located in dark or low-oxygen waters, but little is known of the seep characteristics and the mat communities in shallow, oxygen-rich continental shelf environments (Paul et al., 2017; Ruff et al., 2015) such as the seeps encountered during the SEASCAPES project.
Shallow-Water Cold Seep Discovery
At shallow-water depths, such as on continental shelves where light penetrates to the seafloor, chemotrophs must compete with fauna that rely on photosynthetic carbon. Thus, cold seeps in shallow depths must be more productive than the seeps found in the deep ocean for the same abundance of chemotrophs to persist (Ding & Valentine, 2008; Montagna et al., 1987). Therefore, the presence of large bacterial mats observed during the remotely operated vehicle (ROV) dives offshore Anacapa Island indicate that methane is actively being released into the marine environment despite the lack of visible gas in the water column.
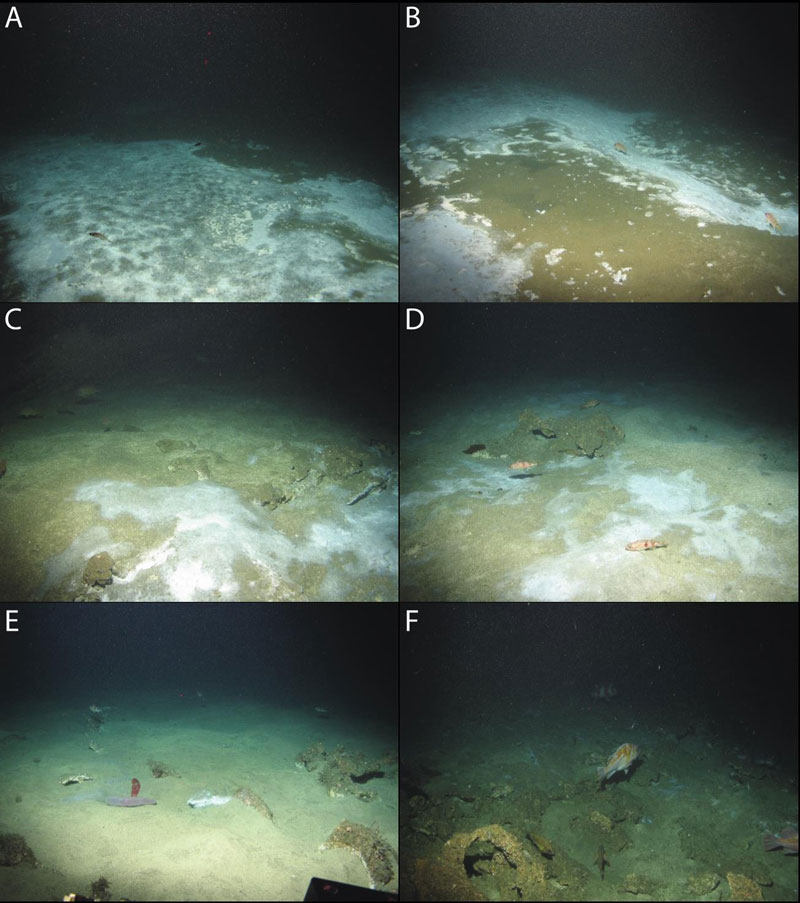
Bacterial mats are a major sink of methane transport into the atmosphere (Paul et al., 2017), so the lack of visible gas bubbles could indicate that most of the methane rising from the sub-seabed is being used by the mats for anaerobic oxidation close to the seabed (Boetius & Wenzhöfer, 2013). The diversity of carbonate structures, ranging from low to high relief, combined with the higher rates of sedimentation on continental shelves, could indicate that these cold seeps have been active for centuries or longer (Levin et al., 2016).
Cold seeps provide habitat for specialized pelagic (water column) and benthic (seafloor) communities that have influence on the marine environment far beyond the footprint of the seep itself. This is because seeps and their sites are both major sources and sinks of global carbon, making these areas key players in global biogeochemical budgets (Levin et al., 2016). Recent estimates show that cold seep flux may account for up to 35% of the world's methane total emissions; but, incredibly, these sites only contribute 1% to 5% of the net methane emissions in the atmosphere due to the bacterial activity, or biological filter, at the seeps sites (Dickens, 2003; Kvenvolden & Rogers, 2005; Milkov et al., 2003). However, these estimates are predominantly derived from global-scale modeling and from site studies in deepwater environments that may not be representative of seep and mat behavior at shallower depths.
The sites discovered during the SEASCAPES project are in shallow water (less than 100 meters (328 feet) depth). The discovered seep field has been given the Sʰamala (Chumashan language) name Ma saputiwaxmu', which means the place where it seeps through, by the Cultural Department at the Santa Ynez Band of Chumash Indians. This tribe is one band of the Chumash people, the original caretakers of the land where this research was conducted.
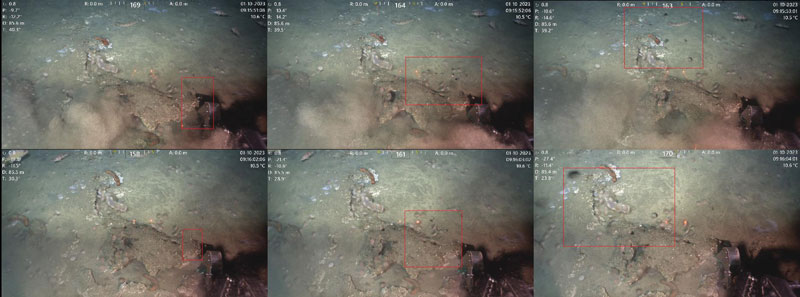
From the ROV footage collected during the final phase of the project, it appears both chemotrophs and phototrophs (organisms such as shallow-water corals that rely on light for production of their primary energy) have taken advantage of the habitat, giving rise to areas with higher biological activity than the surrounding area. This is not unusual, and bacterial mats can become keystone species for the local natural environment (Lv et al., 2022). Geochemical and microbial analyses of samples collected during dives may constrain the activity and metabolic rates of the mats currently present at the seeps. Additionally, offshore Anacapa, the cold seeps provide hard substrate in an area of otherwise predominately soft substrate.
These ecosystems are understudied and recent analysis indicates that many shallow cold seeps are already being impacted by human activity, leaving the local ecosystems vulnerable (Noble-James et al., 2020). Although outside the scope of the SEASCAPES project, the seeps identified offshore Anacapa Island could be used to better the understanding of the communities reliant on the seep activity and thereby minimize the disturbance from human activity. The location of the seep field is easily accessible by a number of research institutions (several of which are already actively involved in cold seep studies elsewhere) in southern California, thereby providing an excellent opportunity for further study of the ecology of cold seeps on continental shelves.
By Dr. Roslynn King, Post-Doctoral Scholar, Scripps Institution of Oceanography
Published June 24, 2024
References
Al-Zaidan, A. S. Y., Kennedy, H., Jones, D. A., & Al-Mohanna, S. Y. (2006). Role of microbial mats in Sulaibikhat Bay (Kuwait) mudflat food webs: evidence from δ13C analysis. Marine Ecology Progress Series, 308, 27–36.
Baker, P. A., & Burns, S. J. (1985). Occurrence and Formation of Dolomite in Organic-Rich Continental Margin Sediments’. AAPG Bulletin, 69(11), 1917–1930.
Boetius, A., & Wenzhöfer, F. (2013). Seafloor oxygen consumption fuelled by methane from cold seeps. In Nature Geoscience (Vol. 6, Issue 9, pp. 725–734). https://doi.org/10.1038/ngeo1926
Dando, P. R. (2010). Biological communities at marine shallow-water vent and seep sites. In The Vent and Seep Biota: Aspects from Microbes to Ecosystems (pp. 333–378). http://www.springer.com/series/6623
Dickens, G. R. (2003). Rethinking the global carbon cycle with a large, dynamic and microbially mediated gas hydrate capacitor. Earth and Planetary Science Letters, 213(3–4), 169–183. https://doi.org/10.1016/S0012-821X(03)00325-X
Ding, H., & Valentine, D. L. (2008). Methanotrophic bacteria occupy benthic microbial mats in shallow marine hydrocarbon seeps, Coal Oil Point, California. Journal of Geophysical Research: Biogeosciences, 113(1). https://doi.org/10.1029/2007JG000537
Elvert, M., Suess, E., & Whiticar, M. J. (1999). Anaerobic methane oxidation associated with marine gas hydrates: superlight C-isotopes from saturated and unsaturated C 20 and C 25 irregular isoprenoids. In Naturwissenschaften (Vol. 86). Springer-Verlag.
Hinrichs, K. U., Hayes, J. M., Sylva, S. P., Brewer, P. G., & DeLong, E. F. (1999). Methane-consuming archaebacteria in marine sediments. Nature, 398(6730), 802–805.
Jessen, G. L., Pantoja, S., Gutiérrez, M. A., Quiñones, R. A., González, R. R., Sellanes, J., Kellermann, M. Y., & Hinrichs, K. U. (2011). Methane in shallow cold seeps at Mocha Island off central Chile. Continental Shelf Research, 31(6), 574–581. https://doi.org/10.1016/j.csr.2010.12.012
Kvenvolden, K. A., & Rogers, B. W. (2005). Gaia’s breath - Global methane exhalations. Marine and Petroleum Geology, 22(4 SPEC. ISS.), 579–590. https://doi.org/10.1016/j.marpetgeo.2004.08.004
Levin, L. A., Baco, A. R., Bowden, D. A., Colaco, A., Cordes, E. E., Cunha, M. R., Demopoulos, A. W. J., Gobin, J., Grupe, B. M., Le, J., Metaxas, A., Netburn, A. N., Rouse, G. W., Thurber, A. R., Tunnicliffe, V., Van Dover, C. L., Vanreusel, A., & Watling, L. (2016). Hydrothermal vents and methane seeps: Rethinking the sphere of influence. Frontiers in Marine Science, 3(MAY). https://doi.org/10.3389/fmars.2016.00072
Lv, Y., Yang, S., Xiao, X., Zhang, Y., María, E., & Zambrano, M. (2022). Stimulated Organic Carbon Cycling and Microbial Community Shift Driven by a Simulated Cold-Seep Eruption. https://journals.asm.org/journal/mbio
Milkov, A. V., Sassen, R., Apanasovich, T. V., & Dadashev, F. G. (2003). Global gas flux from mud volcanoes: A significant source of fossil methane in the atmosphere and the ocean. Geophysical Research Letters, 30(2). https://doi.org/10.1029/2002GL016358
Montagna, P. A., Bauer, J. E., Toal, J., Hardin, D., & Spies, R. B. (1987). Temporal variability and the relationship between benthic meiofaunal and microbial populations of a natural coastal petroleum seep. Journal of Marine Research, 45, 761–789.
Noble-James, T., Judd, A., Diesing, M., Clare, D., Eggett, A., Silburn, B., & Duncan, G. (2020). Monitoring shallow methane-derived authigenic carbonate: Insights from a UK Marine Protected Area. Aquatic Conservation: Marine and Freshwater Ecosystems, 30(5), 959–976. https://doi.org/10.1002/aqc.3296
Paul, B. G., Ding, H., Bagby, S. C., Kellermann, M. Y., Redmond, M. C., Andersen, G. L., & Valentine, D. L. (2017). Methane-oxidizing bacteria shunt carbon to microbial mats at a marine hydrocarbon seep. Frontiers in Microbiology, 8(FEB). https://doi.org/10.3389/fmicb.2017.00186
Ruff, S. E., Biddle, J. F., Tesked, A. P., Knittel, K., Boetius, A., & Ramette, A. (2015). Global dispersion and local diversification of the methane seep microbiome. Proceedings of the National Academy of Sciences of the United States of America, 112(13), 4015–4020. https://doi.org/10.1073/pnas.1421865112
Thiel, V., Peckmann, J. J., Seifert, R., Wehrung, P., Reitner, J., & Michaelis, W. (1999). Highly isotopically depleted isoprenoids: Molecular markers for ancient methane venting.
Tunnicliffe, V., Juniper, S. K., & Sibuet, M. (2003). Reducing environments of the deep-sea floor. In P. A. Tyler (Ed.), Ecosystems of the Deep Oceans (pp. 81–110). Elsevier.
Van Dover, C. L., & Fry, B. (1994). Microorganisms as food resources at deep‐sea hydrothermal vents. Limnology and Oceanography, 39(1), 51–57. https://doi.org/10.4319/lo.1994.39.1.0051